Update
We recently advanced our techniques in in the field of micro-manipulation:
- combining laser-induced flow with microfluidics to achieve unprecedented control in the assembly and operation of micro-robots and a new level of non-invasive manipulation of the nucleus
Opto-fluidically Multiplexed Assembly and Micro-robotics
This research paper introduces a groundbreaking method in the field of micro-manipulation, combining laser-induced flow with microfluidics to achieve unprecedented control in the assembly and operation of micro-robots. Unlike traditional optical tweezers, this technique does not depend on the material properties of the particles and avoids excessive laser exposure. By leveraging multiplexed temperature stimuli, it enables the precise and accelerated positioning of micro-particles, transcending previous limitations of microfluidic technologies. This innovative approach not only enhances capabilities in micro-manufacturing and life sciences but also pioneers the use of opto-hydraulically actuated micro-factories and robotic systems that can express personal characteristics.
Probe-free Optical Chromatin Deformation and Measurement of Differential Mechanical Properties in the Nucleus
This study presents an innovative technique for exploring the mechanical properties of the nucleus, essential for understanding its spatial organization and gene transcription activities. By applying localized temperature gradients, the research achieves significant chromatin displacements within the nucleus without altering its overall structure. Utilizing particle image velocimetry, the team provides detailed strain maps, revealing the distinct mechanical identities of nuclear compartments. This method demonstrates how chromatin's response to mechanical stress can inform on its role in nuclear organization and has potential applications in fields ranging from molecular biology to the development of new therapeutic strategies.
Controlling the physical motion that shapes cells and organisms
Explaining the spatial organization of cells and developing embryos remains a tough nut to crack.
Certainly, we know the rules by which genomic information is translated into proteins, and genetic perturbations have revealed detailed insights into biochemical reaction networks in-vivo.
However, quantitative biophysical models that translate molecular reaction rates (in units of seconds) into length scales (in units of meters), frequently remain at the level of untestable hypotheses. In other words, while biologists rightly demand to test hypothesized reaction networks by genetic perturbations, we largely still lack suitable experimental tools to test the role of physics in patterning, morphogenesis and spatially distributed information processing.
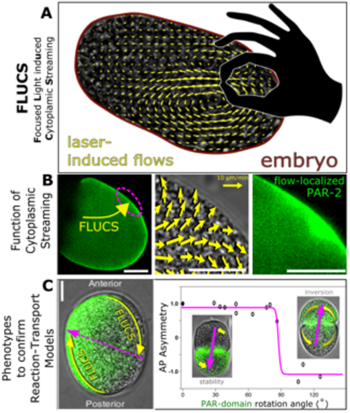
And clearly, how would one change direction, velocity or temporal persistence of cytoplasmic flows in a developing embryo to resolve the question of what they are good for? This is evidently hard to achieve by genetics. As a result of this methodological shortcoming, there are only extremely few accepted proofs of reaction-transport systems in biology.
Recently, we succeeded in optically generating hydrodynamic flows within living cells and developing embryos via thermo-viscous expansion phenomena (see Figure 1a, Mittasch et al., Nature Cell Biology 2018, find video summary here: https://tinyurl.com/yaa9lh8c).
This new paradigm of interactive microscopy in biology is called Focused Light induced Cytoplasmic Streaming (FLUCS). FLUCS is localized, directed, dynamic, physically tractable, and non-invasive. Using FLUCS in early embryos of the nematode worm C. elegans, we revealed that i) cytoplasmic streaming accelerates the zygote’s PAR polarization via directional protein transport (Fig 1b), ii) we gained control over cortical flows (not shown), and iii) proved sufficiency of cortical flows to transport the polarization defining PAR proteins, a key hypothesis in the field of developmental biology (Munro et al, 2004). iv) Guided by FEM simulations we further managed to invert the biochemically-defined head-to-tail body axis of embryos. Most strikingly, flow-induced phenotypes revealed that body axis formation is a bi-stable process (Fig 1c). For its potential in cell and developmental research see also featuring articles about our FLUCS method in Nature Cell Biology and Nature Methods, and our perspective article (Kreysing, Developmental Cell, 2019). Accordingly, with the Grill and Jülicher labs, we recently used FLUCS to control oocyte growth in worms (Chartier et al, Nature Physics 2021).
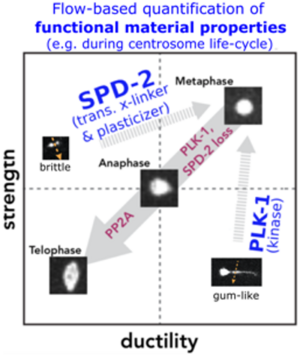
Moreover, we used FLUCS to measure functional material properties of cellular constituents in-vivo, e.g. to confirm a metabolically induced fluid-to solid transition of the yeast cytoplasm (collaboration with the Alberti lab, Dresden), and to dissect the molecular pathways underlying cell cycle dependent material properties of the centrosome in developing worms (collaboration with the Woodruff lab, Medical Center UTSW, Mittasch et al, J Cell Biol 2020, figure r.h.s.).
Lately, we are working to increase the precision of flow perturbations for particle positioning (currently up to 25nm, Erben et al, Optics Express (2021), sub-nuclear manipulations and laser exposure free force measurements with femtoNewton sensitivity (Stoev et al, eLight 2021).
In collaborations, our well-defined and localised temperature perturbations have further been shown to be instrumental to dissect the physics of phase separation in vivo (Fritsch et al, PNAS 2021; Iserman et al, Cell 2020; Saito et al, Nat Chem Biol 2019).
To meet the communities responses, we made FLUCS technology commercially available with our industrial collaborator Rapp Opotoelectronic GmbH.
https://rapp-opto.com/products/photomanipulation-systems/flucs-micro-flow-photomanipulation/
GHOSTs (Genetically enhanced optically superior tissues, ERC funded)
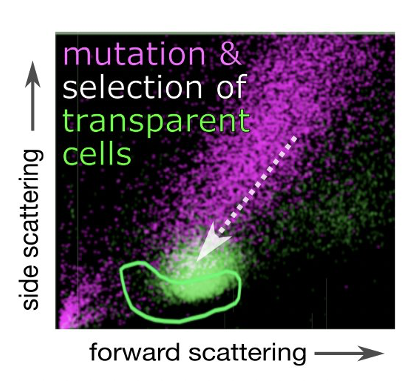
Most biological tissues are optically opaque, largely precluding access by light microscopy.
In stark contrast, some living tissues and organisms are highly transparent. Examples include many deep-sea fish, your retina, and cultured mammalian cells that we exposed to directed evolution (Subramanian et al, J Biophoton 2020, see image below).
Supported by an ERC grant (GHOSTs), we now set out to uncover the genetic basis of tissue transparency, such that living cells and tissue cultures can be optically cleared by precision genetics. For this we combine insights into the origins of retina transparency (Solovei et al, Cell 2009, Subramanian et al, eLife 2019), and a unique set of methods, to answer the question how genetically cells become more transparent during directed evolution.
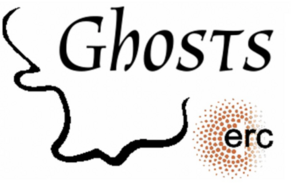
Knowing about physiological transparency genes will allow unprecedented insights into living tissues. If model tissues in the lab were just 1% as transparent as some glass-like fish found in the deep sea, optical microscopes could unleash their full potential, and enable high resolution views into developmental processes in their native environment. We see further transformative potential especially in the fields of organotypic tissue models, functional brain imaging, as well as pharmaceutical screens in 3D tissue cultures.
The origin of life (VW foundation funded)
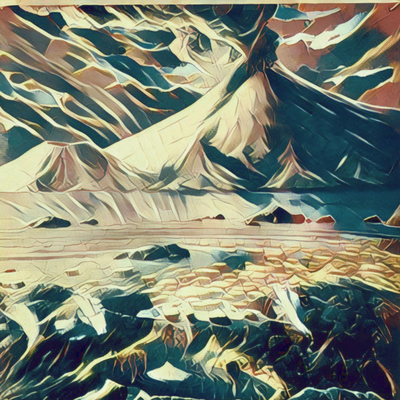
The most remarkable aspect of life is its mysterious origin.
Although chemists are now able to create the building blocks of life in the lab, the magic spark that makes these molecules self-assemble into something living could not be observed yet. And indeed, a basic law of physics dictates that man made constructs are prone to decay when left to themselves. Our past insights reveal that first replicating entities on our planet were not entirely alone though, but taken care of by heat driven micro-reactors inside submarine volcanic rocks. In these little compartments genetic molecules get trapped and find optimal conditions for replication. Additionally they are selected towards ever increasing complexity, reversing the usual one-way route from living organisms to dead matter.
Reference: Moritz Kreysing, Lorenz Keil, Simon Lanzmich, and Dieter Braun
Nature Chemistry (2015) doi:10.1038/nchem.2155
More recently, we found that also phase separated protocells can be assembled in this setting, and we are studying the implications of this finding supported by a 5-year collaborative grant that we received from the Volkswagen Foundation. Such phase-separated compartments have long been proposed as precursors to cellular life. Now, it we have shown that RNA–peptide protocells are more robust when formed using shorter (rather than longer) peptides, and that peptide sequence determines the functional materials properties of these compartments.
Reference: Juan M. Iglesias-Artola, Björn Drobot, [...], Moritz Kreysing
Nature Chemistry (2022) doi.org/10.1038/s41557-022-00890-8